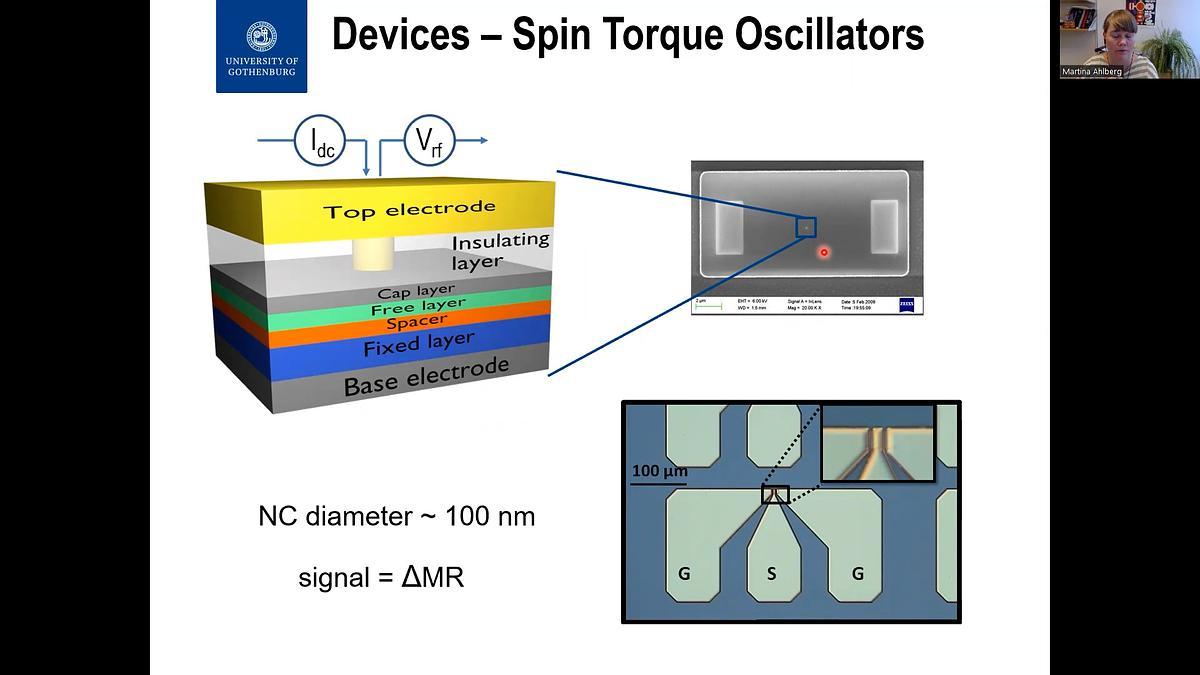
Premium content
Access to this content requires a subscription. You must be a premium user to view this content.
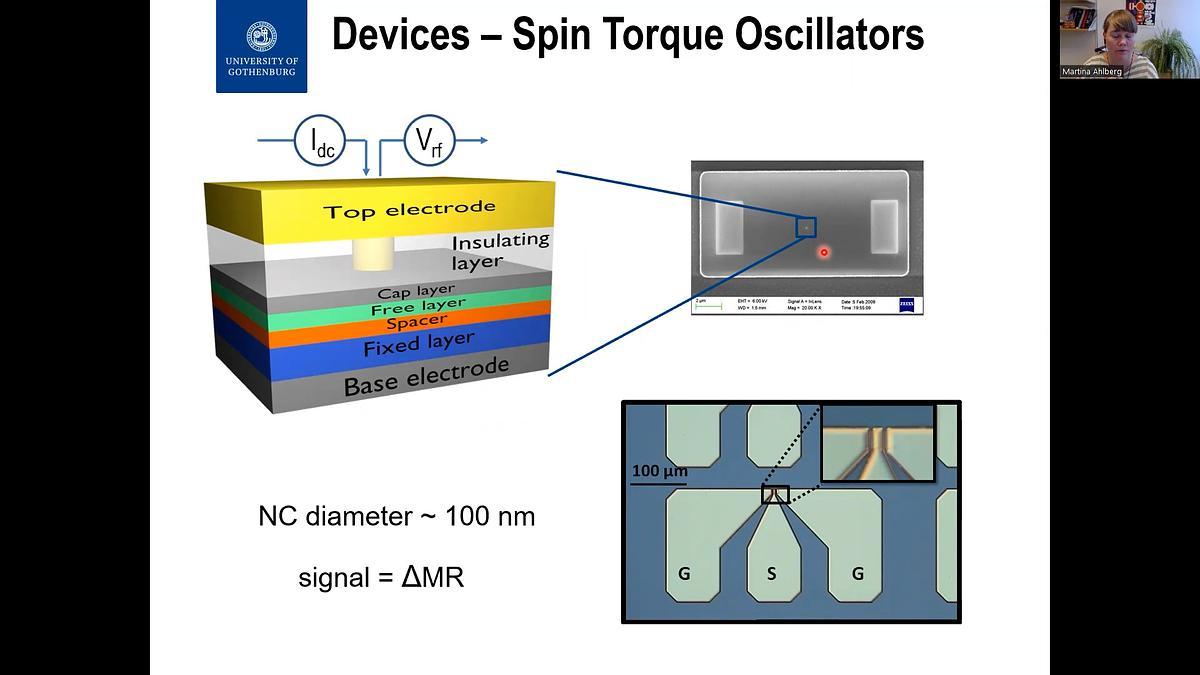
technical paper
Freezing and thawing magnetic droplet solitons
We use nanocontact spin-torque oscillators (STNOs) to explore the applied field and current dependence of magnetic droplets. These dissipative solitons are found in
magnetic layers with perpendicular anisotropy, and they are inherently dynamic and characterized by a core of reversed spins surrounded by a precessing perimeter 1, 2, 3, 4. The
precession frequency lies between the ferromagnetic and Zeeman resonances, but the droplet is also prone to drift which gives additional dynamics 5, 6. Orthogonal pseudo-spin-valves
are usually used in experiments to harvest a strong magnetoresistive dynamic signal 2, 3, 5, 6, but here we use an all-perpendicular layout to study the otherwise inaccessible low field
region.
Electrical measurements reveal that the droplet transforms, freezes, into a static bubble at low fields. Figure 1 illustrates the electrical characteristics of the different states. The resistance is
similar for the droplet and the bubble (Fig. 1a), but the difference in noise level is striking (Fig. 1b) and demonstrates the bubble’s static nature. Once formed, the bubble is stable without a
sustaining current. Furthermore, the droplet-to-bubble transition is fully reversible, and the bubble can thaw back to a droplet at sufficient high field and current. The findings are
corroborated by X-ray microscopy, which images the magnetic states during the freezing. Experimental data together with simulations identify pinning as the main mechanism behind the
bubble stability.
References
1 Hoefer et al., Theory for a dissipative droplet soliton excited by a spin torque nanocontact Phys. Rev. B 82, 054432 (2010)
2 Mohseni et al., Spin Torque–Generated Magnetic Droplet Solitons Science 339, 1295 (2013)
3 Chung et al., Spin transfer torque generated magnetic droplet solitons (invited) J. Appl. Phys 115, 172612 (2014)
4 Chung et al., Direct Observation of Zhang-Li Torque Expansion of Magnetic Droplet Solitons Phys. Rev. Lett. 120, 217204 (2018)
5 Xiao et al., Parametric autoexcitation of magnetic droplet soliton perimeter modes Phys. Rev. B 95, 024106 (2017)
6 Chung et al, Magnetic droplet nucleation boundary in orthogonal spin-torque nano-oscillators Nature Com. 7, 11209 (2016)
Figure 1: Phase diagrams based on the resistance and the microwave noise. (a) STNO resistance and (b) integrated (0–0.5 GHz) microwave noise level as a function of field and current. (c)
shows the noise level in (b) overlaid on the resistance in (a) displayed using a gray scale highlighting intermediate resistance levels indicative of droplets/bubbles. The parallel (P) and
antiparallel (AP) states are easily discernible in the MR-map (a) as dark blue and dark red, while both the droplet and the bubble are characterized by intermediate resistance in green–
yellow. The stark difference between the droplet and the bubble is revealed in the noise spectrum (b), where the stability of the bubble is manifested. Note however that the light-blue
flanges in (a) correspond to a different droplet regime not captured in the microwave signal presented in (b).