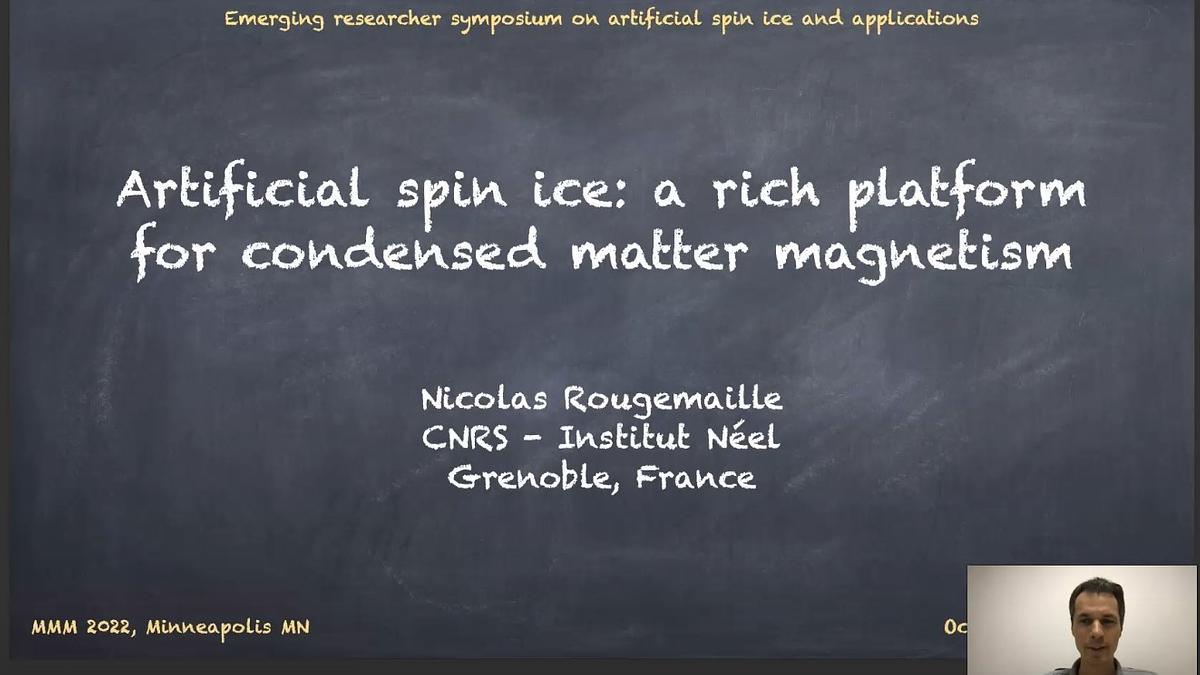
Premium content
Access to this content requires a subscription. You must be a premium user to view this content.
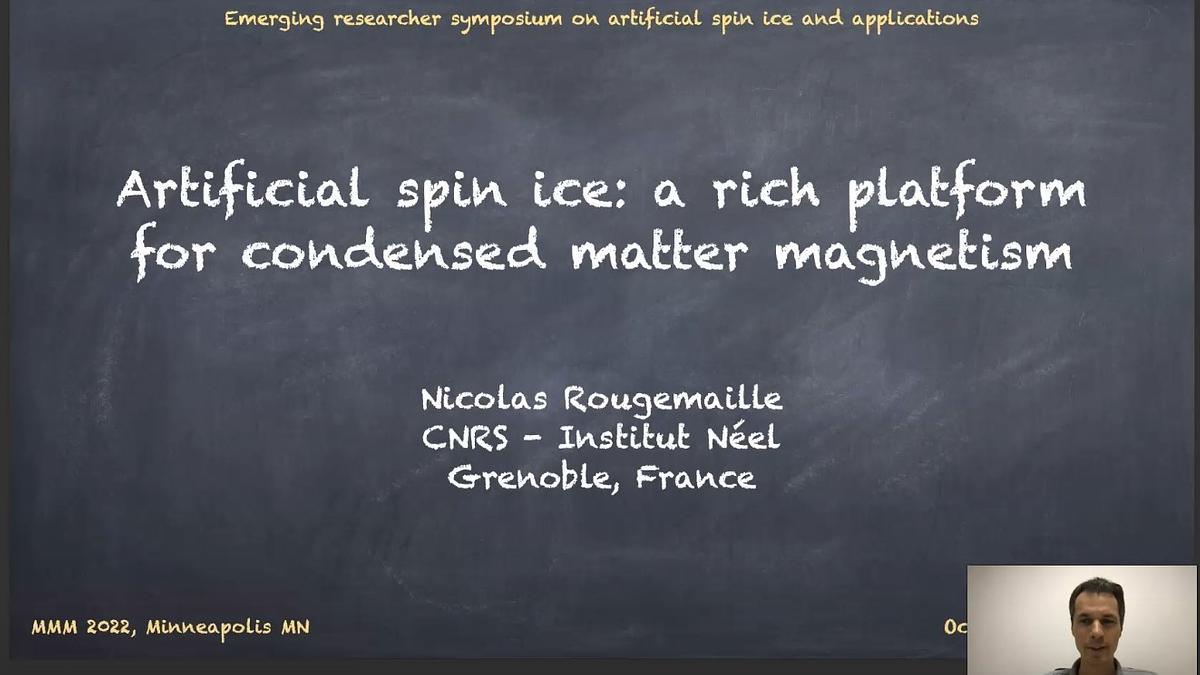
technical paper
Artificial spin ice: a rich platform for condensed matter magnetism
Artificially made, geometrically frustrated arrays of interacting magnetic nanostructures offer a remarkable playground for exploring spin ice physics experimentally 1-3. Initially designed to capture the low-energy properties of highly frustrated magnets 4,5, artificial spin ices became in the past decade a powerful lab-on-chip platform in which to investigate cooperative magnetic phenomena and exotic states of matter. Since their introduction about fifteen years ago, the interest in artificial spin ice systems largely broadened, and the term now covers a wide range of physical phenomena and systems extending well beyond artificial ice-like magnets. Besides fundamental works, many studies were also conducted recently on the applicability of artificial spin ice geometries in magnetic architectures, in which the micromagnetic degree of freedom, spin wave excitations or topological magnetic pseudo-charges for example are envisioned as carriers for information transfer, storage and processing 6-8. The richness of this fast evolving research field is certainly the variety of expertises and scientific backgrounds gathered within a single community, with scientists working in different, sometimes separated, areas of condensed matter magnetism, spreading from nanomagnetism and spintronics to frustrated magnetism and statistical mechanics. In this presentation, recent advances in the field will be overviewed and potential routes for future works will be discussed.
References:
1 C. Nisoli, R. Moessner and P. Schiffer, Rev. Mod. Phys. 85, 1473 (2013).
2 N. Rougemaille and B. Canals, Eur. Phys. J B 92, 62 (2019).
3 S. H. Skjærvø, C. H. Marrows, R. L. Stamps and L. J. Heyderman Nat. Rev. Phys. 2, 13 (2020).
4 M. Tanaka et al., J. Appl. Phys. 97, 10J710 (2005); Phys. Rev. B 73, 052411 (2006).
5 R. F. Wang et al., Nature 439, 303 (2006).
6 S. Gliga, E. Iacocca and O. G. Heinonen, APL Mater. 8, 040911 (2020).
7 M. T. Kaffash, S. Lendinez and M. B. Jungfleisch, Phys. Lett. A 402, 127364 (2021).
8 J. C. Gartside et al., Nat. Nanotech. 17, 460 (2022).