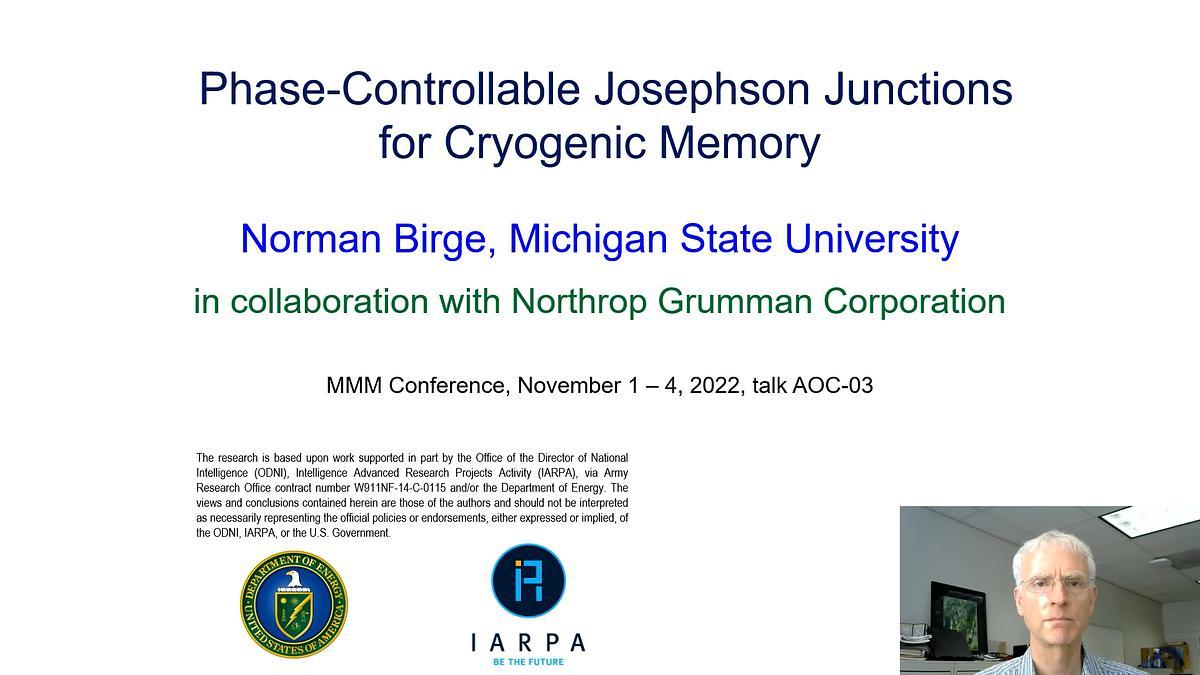
Premium content
Access to this content requires a subscription. You must be a premium user to view this content.
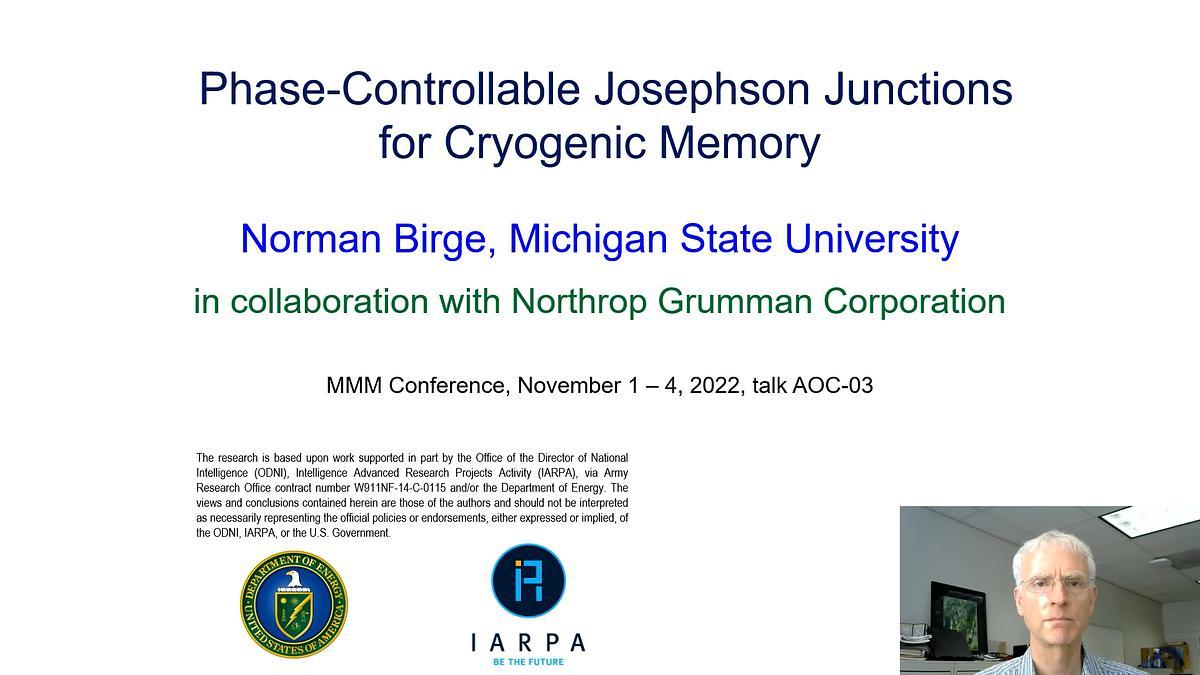
technical paper
Ferromagnetic Josephson Junctions for Cryogenic Memory
Interest in superconducting computing is being driven by two concerns. On the one hand, large-scale computing facilities and data centers are using electrical power at an ever-increasing rate. Projections suggest that a fully superconducting computer would consume considerably less power than conventional semiconductor-based computers 1. On the other hand, a quantum computer based on superconducting qubits would benefit from having a classical superconducting processor nearby in the cold space, to avoid a plethora of control and readout lines between room temperature and the cold space 2. Building a large-scale memory for a superconducting computer is a challenge. One approach is to use Josephson junctions containing ferromagnetic materials as the basic memory element for such a memory 3. In that approach, the information storage device is a Josephson junction containing a pseudo spin valve. Depending on the relative orientation of the two magnetizations in the spin valve, and with suitably chosen thicknesses of both magnetic layers, the ground-state phase difference across the junction can be either zero or π. We have demonstrated two different types of junctions that can be controllably switched between the 0-phase state and the π-phase state 4,5. While such junctions are promising components for a scalable superconducting memory 6, much more work needs to be done to enable scaling of this approach to a large-scale memory array.
Work supported by the US DOE under grant DE-FG02-06ER46341, by Northrop Grumman Corporation, and by IARPA via U.S. Army Research Office contract W911NF-14-C-0115. The views and conclusions contained herein are those of the authors and should not be interpreted as necessarily representing the official policies or endorsements, either expressed or implied, of the ODNI, IARPA, or the U.S. Government.
References
1 D.S. Holmes, A.L. Ripple, & M.A. Manheimer, IEEE Trans. Appl. Supercond. 23, 1701610 (2013).
2 E. Leonard et al., Phys. Rev. Applied 11, 014009 (2019).
3 A.Y. Herr & Q.P. Herr, US Patent 8,270,209 (2012).
4 E. C. Gingrich, B. M. Niedzielski, J. A. Glick, Y. Wang, D. L. Miller, R. Loloee, W. P. Pratt Jr., and N. O. Birge, Nature Phys. 12, 564 (2016).
5 J.A. Glick, V. Aguilar, A. Gougam, B.M. Niedzielski, E.C. Gingrich, R. Loloee, W.P. Pratt, Jr., and N.O. Birge, Science Advances 4, eaat9457 (2018).
6 I. M. Dayton et al., IEEE Magnetics Lett. 9, 3301905 (2018).