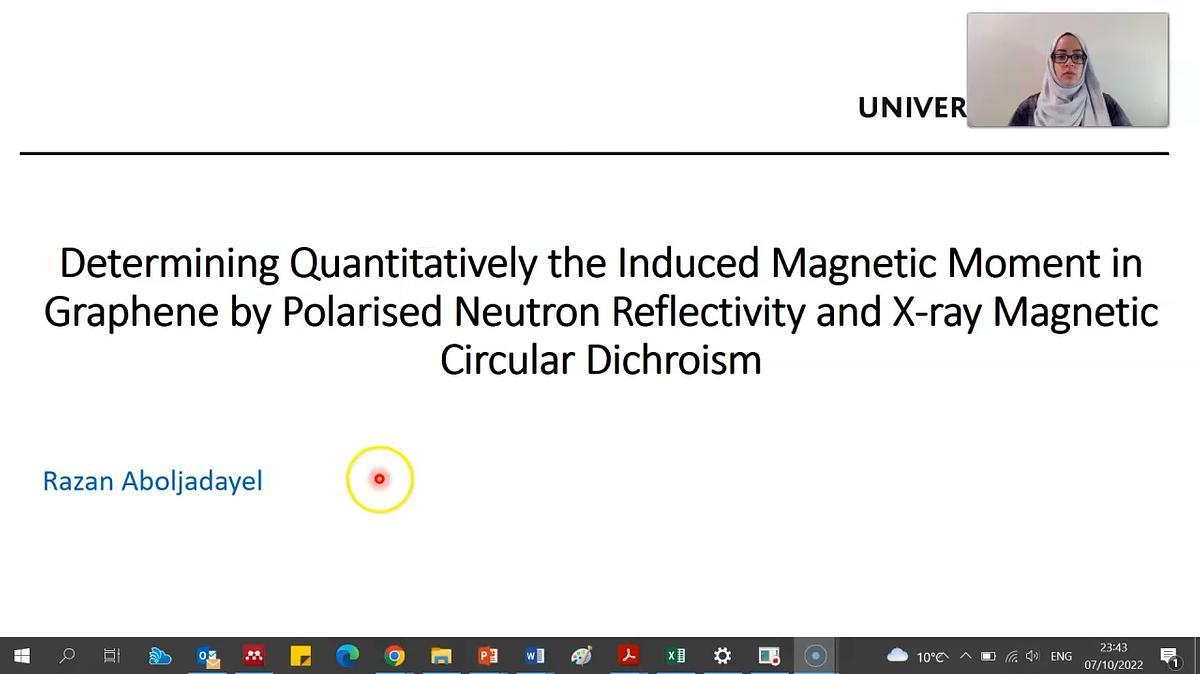
Premium content
Access to this content requires a subscription. You must be a premium user to view this content.
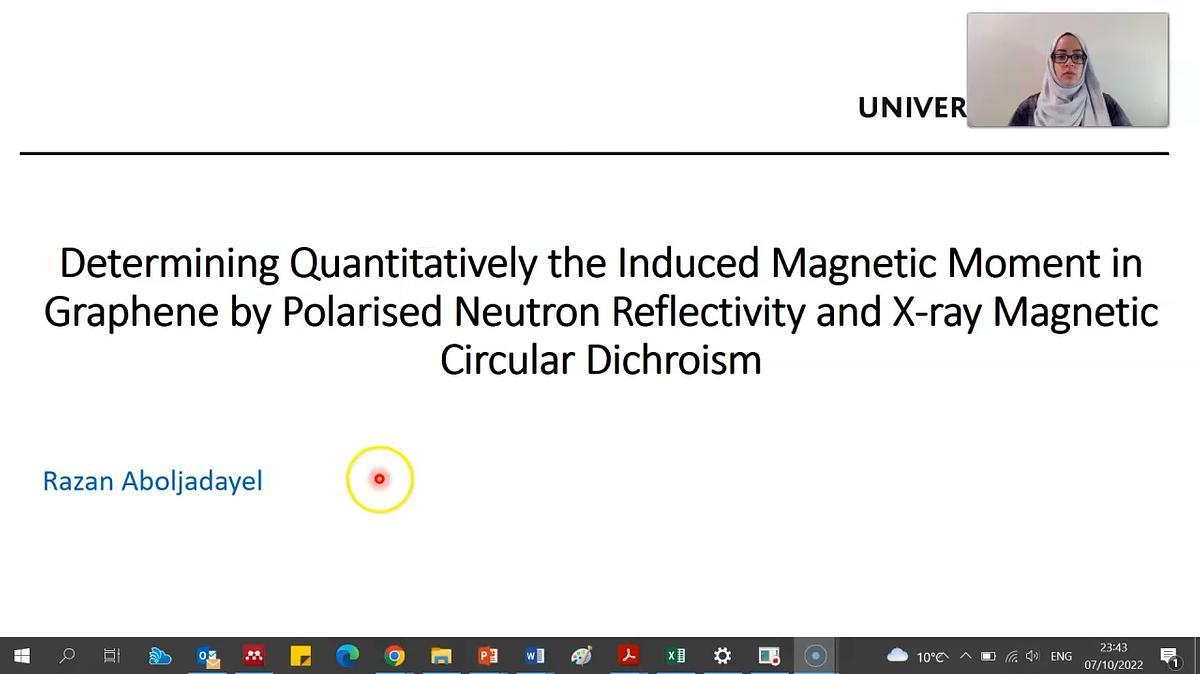
technical paper
Determining Quantitatively the Induced Magnetic Moment in Graphene by Polarised Neutron Reflectivity and X ray Magnetic Circular Dichroism
Graphene has unique electronic properties which make it a promising material for next-generation electronics. It has high electron mobility at room temperature and a long spin-diffusion length. The latter is due to its weak intrinsic hyperfine interactions and small spin-orbit coupling. Therefore manipulating spins directly in a layer of pristine graphene is not easy. However, this can be overcome by an induced proximity effect using an exchange-coupled interface between the graphene and a ferromagnetic layer. Several studies predicted that the conduction band of graphene will spin split at the interface with a ferromagnetic substrate 1-4.
We report the magnitude of the induced magnetic moment in graphene as a result of the proximity effect in the vicinity of a Ni layer using polarised neutron reflectivity (PNR) and X-ray magnetic circular dichroism (XMCD). The Ni film was grown by sputtering upon which the graphene was grown by chemical vapour deposition. The growth parameters were tuned to produce epitaxial and rotated graphene domains (Fig. 1). The XMCD results at the C K-edge confirm the presence of magnetic polarisation in rotated graphene. Intensive quantitative analysis of the PNR fits, ascertained based on Bayesian analysis, indicate that at 10 K 0.53 and 0.38 μB/C atom is induced in rotated and epitaxial graphene grown on Ni(111), respectively. These values are three times higher than those predicted in other studies 4,5. Two possible mechanisms were elaborated for the observed induced magnetic moment 4,6,7. To clarify the origin of the measured moment, further PNR measurements were carried out on graphene grown on a non-magnetic substrate (Ni9Mo1) 8.
We will present the different models used to fit the data and discuss the challenges of fitting the PNR of a 2D material which is within the resolution limit of the technique. We will also show the results of other techniques used to characterise the samples.
References:
1 H. Haugen et al., Phys. Rev. B 77, 115406 (2008).
2 Q. Zhang et al., Appl. Phys. Lett. 98, 032106 (2011).
3 H.X. Yang et al., Phys. Rev. Lett. 110, 046603 (2013).
4 M. Weser, Y. Rehder et al., Appl. Phys. Lett. 96, 012504 (2010).
5 H. -Ch. Mertins et al., Phys. Rev. B 98, 064408 (2018).
6 V. Karpan, P. Khomyakov et al., Phys. Rev. B 78, 195419 (2008).
7 F. Ma’Mari, T. Moorsom et al., Nature 524, 69 (2015).
8 R. O. M. Aboljadayel et al., arXiv:2101.09946v2 (2022).
SEM images at 1 kV showing the graphene domains for (a) epitaxial graphene/Ni and (b) rotated graphene/Ni and (c) The LEED diffraction pattern of epitaxial graphene on a Ni(111) substrate at 300 eV. The red circle in (b) highlights a single graphene domain with a diameter of ∼ 0.25 μm.