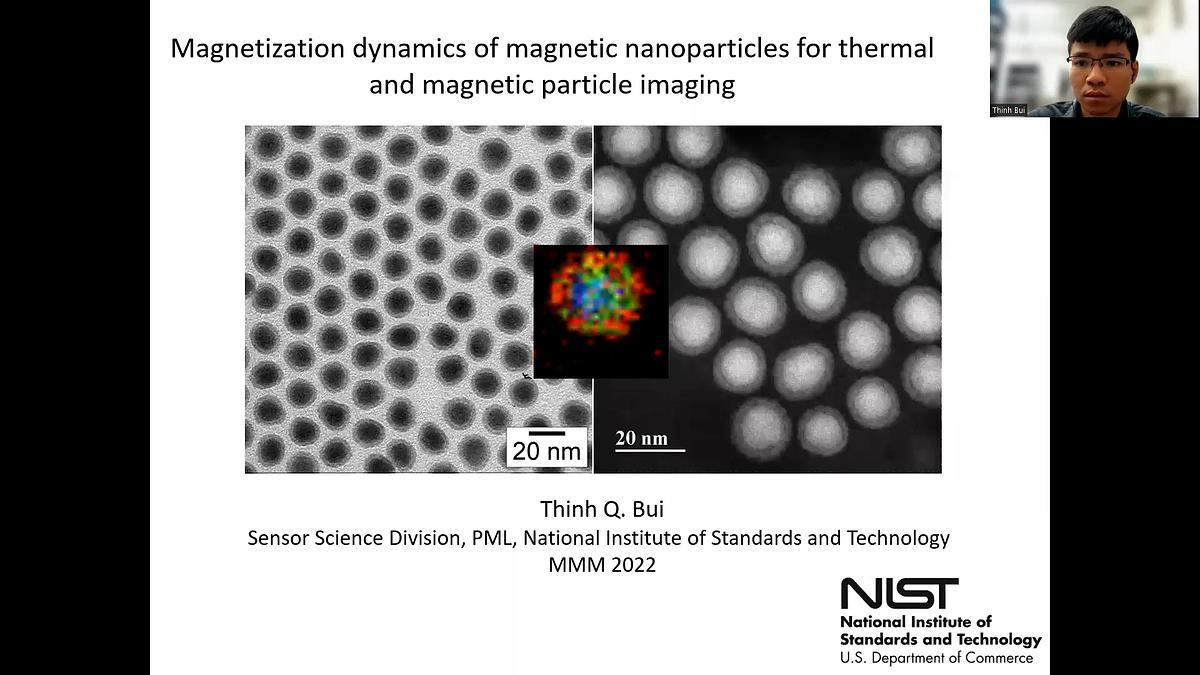
Premium content
Access to this content requires a subscription. You must be a premium user to view this content.
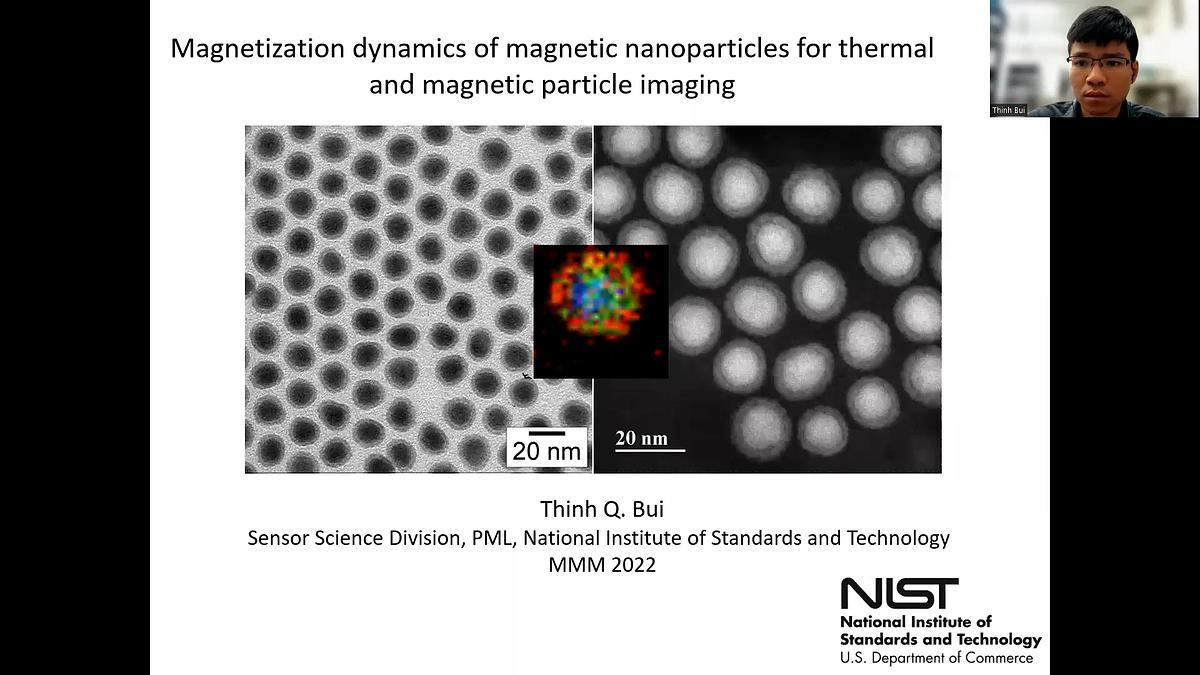
technical paper
Coupled Néel Brown magnetization dynamics of magnetic nanoparticles for thermal and magnetic particle imaging
The prospect of thermal imaging and control in magnetic particle imaging (MPI) is an important advancement for non-invasive medical diagnostics and therapeutics.
Current MPI systems focus mainly on imaging from measurement of nanoparticle concentrations. Accurate derivation of temperature relies on detailed understanding of magnetization
dynamics in the presence of AC drive fields and variable temperature 1. It is well established that MPI performance is critically impacted by nanoparticle relaxation dynamics 2. The
dynamic response of magnetic nanoparticles in magnetic fields depends strongly on the particles’ magnetic and structural properties, inter-particle interactions, and the local environment. We report an arbitrary-wave magnetic particle spectrometer for characterizing dynamics over the 200 K to 350 K temperature range. These measurements can separately identify features of
Brownian and Néel relaxation and quantify the timescales and amplitudes associated with these processes.
Here, we discuss measurements of magnetization dynamics using AC susceptibility and pulsed excitation for ferrite and cobalt-doped ferrite nanoparticles ranging in diameter from 7 nm to
70 nm. For example, Fig. 1 show the imaginary component, χ’’, peak frequency for two different sizes of ferrite nanoparticles 3. Fig. 2 shows the time domain measurement of 7 nm
cobalt-doped ferrite under pulsed excitation in which both field-on and field-free relaxation are observed and quantified. Frequency and time domain measurements for cobalt-doped
ferrites show the presence of both Néel and Brownian dynamics, and the temperature dependence reveals their intrinsic coupling. To resolve this coupling, we used stochastic Monte Carlo
methods to simulate the field and temperature dependence of magnetization dynamics. This knowledge will eventually inform strategies for design of magnetic nanoparticles with
properties targeted for accurate and sensitive thermal imaging using MPI.
References
1 Thinh Q. Bui, Weston L. Tew, Solomon I. Woods. “AC magnetometry with active stabilization and harmonic suppression for magnetic nanoparticle spectroscopy and
thermometry.” J. Appl. Phys. 128, 224901 (2020).
2 Zhi Wei Tay, Daniel W Hensley, Erika C Vreeland, Bo Zheng, Steven M Conolly. “The Relaxation Wall: Experimental Limits to Improving MPI Spatial Resolution by Increasing
Nanoparticle Core size.” Biomed Phys. Eng. Express, 3 (3), 035003 (2017)
3 Thinh Q. Bui, Adam J. Biacchi, Cindi L. Dennis, Weston L. Tew, Angela R. Hight Walker, Solomon I. Woods. “Advanced characterization of magnetization dynamics in iron oxide
magnetic nanoparticle tracers.” Applied Physics Letters, 120, 012407 (2022).
Fig. 1: ACS spectrum of 15 and 20 nm ferrites. The real (χ’) imaginary (χ”) parts, and fits (blue) using a semi-empirical analytical model.
Fig. 2: Magnetic field (~ 5 mT) with 20 ns rise time (blue). Magnetization step-response of 7 nm cobalt-doped ferrite (red).