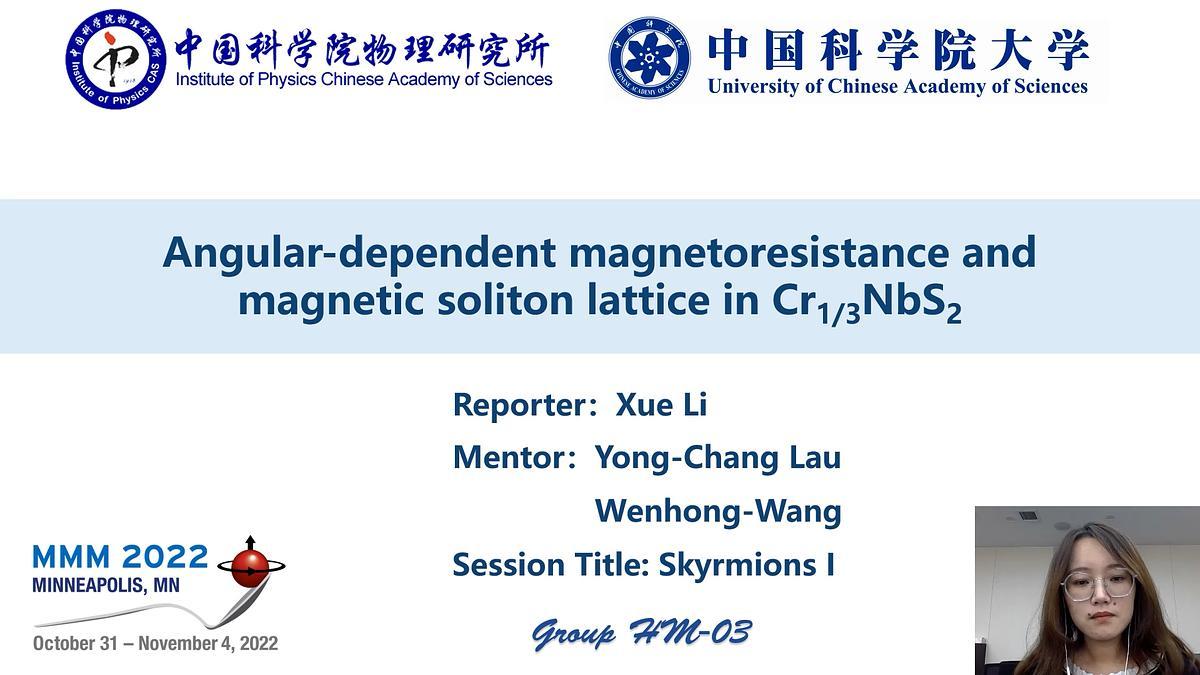
Premium content
Access to this content requires a subscription. You must be a premium user to view this content.
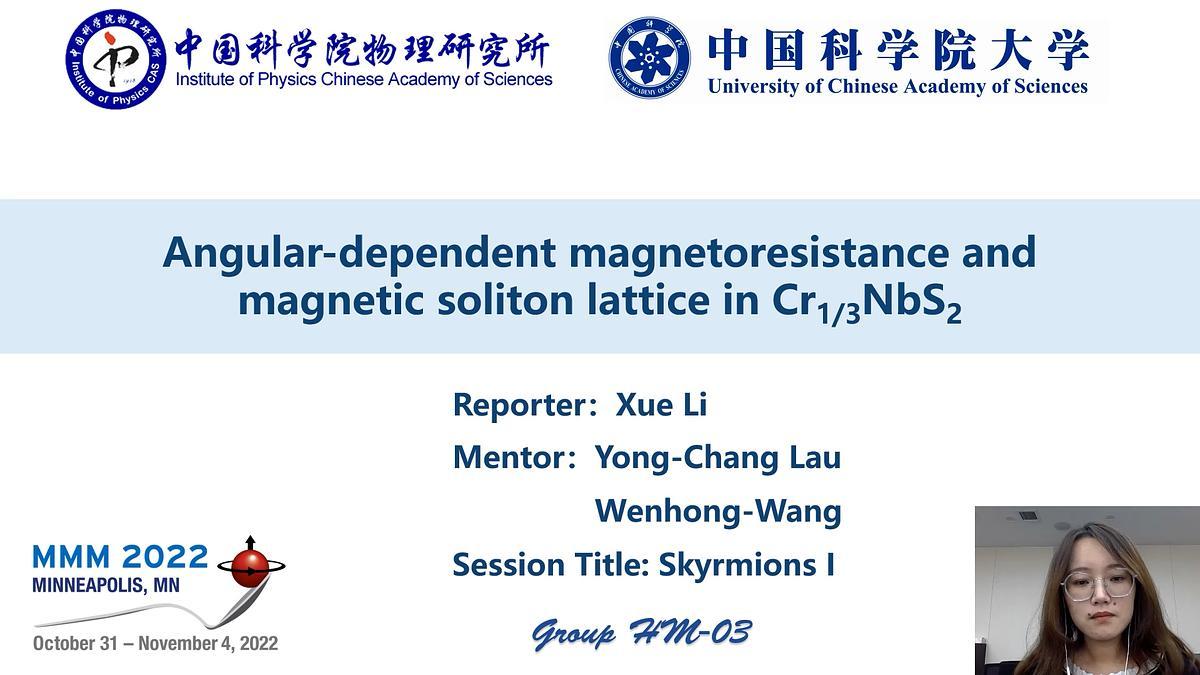
technical paper
Angular dependent magnetoresistance and magnetic soliton lattice in Cr1/3NbS2
Cr1/3NbS2, the first known magnetic soliton system, has abundant magnetic phases like chiral helimagnetic order (HM), chiral conical phase, chiral soliton lattice (CSL), tilted chiral soliton lattice and forced ferromagnetic (FM) due to the competition between Dzyaloshinskii–Moriya exchange, Heisenberg interaction, magnetocrystalline anisotropy and Zeeman energy (1, 2). These rich magnetic textures display attractive properties, such as magnetocaloric effect (3), topological Hall effect and planar Hall effect (4), etc. Especially, magnetic soliton, a real-space topological magnetic structure like skyrmion, owns topological protection properties and quasi-particle nature which makes Cr1/3NbS2 a promising platform for the spintronic devices (5, 6).
Recently, we have studied the transition of various magnetic structures by magnetoresistance (MR) (7), a.c. magnetic susceptibility (7), and Lorentz transmission electron microscopy (L-TEM). As shown in Fig. 1(a), when a magnetic field was applied along the c-axis (H ‖ c - axis) at low temperatures, we found signatures of weak antilocalization which may originate from the strong spin–orbit coupling of Cr1/3NbS2. When the magnetic field was applied along the ab-plane (H ‖ ab - plane), the transition from HM to CSL and FM can be detected by a.c. magnetic susceptibility (Fig. 1(b)). To better understand CSL, we adopted L-TEM measurement. As shown in Fig. 1(c) and (d), a helical period longer than theoretical calculations (~48 nm) and showing opposite chirality due to grain boundary modulation were observed in some regions on the sample. The reason for the difference in period can be the uneven content of Cr intercalation. This may provide an effective way to regulate the helical period, thus facilitating its practical application.
References:
(1) T. Miyadai, K. Kikuchi, H. Kondo, et al., J. Phys. Soc. Jpn. 52 (4), 1394-1401 (1983).
(2) Y. Togawa, T. Koyama, K. Takayanagi, et al., Phys. Rev. Lett. 108 (10), 107202 (2012).
(3) N. J. Ghimire, M. A. McGuire, D. S. Parker, et al., Phys. Rev. B 87 (10), 104403 (2013).
(4) D. A. Mayoh, J. Bouaziz, A. E. Hall, et al., Phys. Rev. Research 4 (1), 013134 (2022).
(5) Y. Togawa, T. Koyama, Y. Nishimori, et al., Phys. Rev. B 92 (22), 220412 (2015).
(6) L. Wang, N. Chepiga, D. K. Ki, et al., Phys. Rev. Lett. 118 (25), 257203 (2017).
(7) X. Li, Z. Li, H. Li, et al., Appl. Phys. Lett. 120 (11), 112408 (2022).